Introductory Physics: A Model Approach
Cover and Dedication
Cover, dedication and copyright information for Introductory Physics: A Model Approach.
Biography
A biography of Robert Karplus, author of Introductory Physics: A Model Approach.
Foreword
A Foreword to Introductory Physics: A Model Approach by Fernand Brunschwig.
Introduction
Robert Karplus wrote this innovative textbook on physics for non-science students between 1965 and 1969. The book first appeared in 1966 as a preliminary edition, and in 1969 W. A. Benjamin, Inc. published the first edition in hardback. For reasons not connected with the quality of the book, it never got to a second edition and has been out of print for many years. As Karplus says in the Author’s Preface below, “. . . this book is ad-dressed particularly to readers with little scientific or mathematical back-ground: the only requirements are common sense, experience, and reasoning ability.” Writing an understandable physics textbook for readers with little background is a laudable goal – often announced, but seldom achieved. In fact, a large variety of magazines, popular books, and textbooks have been published for this audience since 1969. To read the full introduction, click the button below.
Preface
What is physics? Every introductory physics text tries to answer this question in its own way, and this text is no different. However, this book is addressed particularly to readers with little scientific or mathematical background: the only requirements are common sense, experience, and reasoning ability. To make the subject meaningful to this audience, it was necessary to alter the customary approach of most introductory texts. To read the full Preface by Robert Karplus, click the button below.
Have you ever sorted the books in your library according to their subject matter, only to find a few remaining that “didn’t fit”? In a way, this problem is similar to problems that face a scientist. For example, a scientist collects data on crystals or atomic particles or orbiting planets and must face the fact that some of the data does not fit expectations. Such an experience can be unsettling, but it can also lead to new understanding and insight.
One of the primary objectives of this text is to introduce you to a few of the powerful interpretations of natural phenomena used by the physicist to help organize experience. The text discusses some of these phenomena and the patterns of behavior they exhibit. You, in turn, are asked to examine your own experience for additional data to support or contradict these ideas. Occasionally, an unexpected outcome may compel you to reorganize your thinking. A critical approach to all aspects of the text is in order. To read Chapter 1, click the button below.
You may associate the word “relativity” with mathematical mystery and scientific complexity, yet the basic concept, which we will try to explain in these pages, is simple. The matters of concern in relativity are the position (location) and motion of objects. The basic concept is that position and motion of an object can only be perceived, described, and recognized with reference to (that is, “relative” to) other objects.
When you say, “The physics books are at the left rear of the book store,” you refer the position of the books to the entrance and outline of the store. Objects such as the store entrance, to which position or motion are related, are called reference objects. Several reference objects
used in combination to describe position are said to form a reference frame (or frame of reference), and we speak of the position or motion of the original object relative to the reference frame. If we know the position and motion of an object relative to one reference frame, we might ask about the position and motion relative to a second reference frame. This is the root of the theory of relativity: development of specific mathematical models for relating position and motion as observed relative to one reference frame to position and motion as observed relative to another reference frame. Einstein’s theory of relativity is the most complete theory of these relationships. We will describe some aspects of Einstein’s work in Section 7.3, but we will not go into the mathematical details in this text. To read Chapter 2, click the button below.
The interaction concept is being used more and more widely to explain social and scientific phenomena. At conferences, strong interaction may be evident among some participants, weak interaction among others. At the ocean shore, erosion is caused by the interaction of wind and water with rock. In the laboratory, magnets interact even when they are not touching.
A dictionary provides the following definitions: interact (verb): to act upon each other …; interaction (noun): action upon or influence on each other.
To say that objects interact, therefore, is to say that they have a relationship wherein they jointly produce an effect, which is the result of their action upon each other. In the examples cited above, anger may be the effect caused by strong (and irritating) interaction among the conference participants; crumbling and wearing away is the effect of the interaction of wind and water with rocks; and movement toward one another followed by sticking together is the effect of the interaction of the magnets. To read Chapter 3, click the button below.
Matter and energy are of central concern to the physicist. From our ability to make successful theories has come understanding of the ways in which a system may store energy and how energy may be transferred by interaction of objects or systems with one another. From this understanding has come the extensive and effective utilization of energy that is at the base of modern technology and our civilization.
Everyone forms qualitative concepts of matter and energy as a result of everyday experience. Matter is represented by the solid objects, liquids, and gases in the environment.
Matter is tangible; it is capable of interacting with the human sense organs, and various pieces of matter are capable of interacting with one another. Matter appears to be conserved: if an object is once observed in a certain place and later is not there, you are convinced that it has been removed to another location or that it has been made unrecognizable by changes in its appearance. No one believes that it could be annihilated without a trace remaining. To read Chapter 4, click the button below.
The nature of light and sound has intrigued mankind over the centuries. Light and sound are connected with sight and hearing and are therefore vital sources of information about our environment as well as essential for survival. The control of sound has led to spoken language and music. The use of light has led to written language and the visual arts. Hearing and seeing, sound and light, enable us to communicate with one another and to derive pleasure from the natural world as well as from music and art.
We tend to associate light and sound because both are important for sense perception through interaction-at-a-distance. In this way sight and hearing are different from touch, taste, and smell: these three latter senses depend on physical contact of the sense organ and the material (solid, liquid, or gaseous) to be sensed. Both light and sound originate in so-called primary sources: a candle flame, a lightning flash, and the sun emit light; a bowed or plucked violin string, a thunderclap, and a croaking bullfrog produce sound. Both light and sound interact with detector systems: light with the human eye, photographic film, or a video camera; sound with the human ear or a microphone. Both light and sound, therefore, function as intermediaries interaction-at-a-distance.
Waves on a water surface are such a familiar and expected occurrence that a completely still, glassy pool excites surprise and admiration. You can also observe waves on flags being blown by a strong wind. In this chapter you will be concerned with how waves propagate, what properties are used to describe them, and how waves combine with one another when several pass through the same point in space at the same time. In the wave theory, which was formulated by Christian Huygens during the seventeenth century, the space and time distribution of waves is derived from two assumptions, the superposition principle and Huygens’ Principle. The wave theory is very “economical” in the sense that far-reaching consequences follow from only these two assumptions.
In this chapter we will return to the discussion of sound and light that we began in Chapter 5. This time we will describe both phenomena from the point of view of the wave model. As we already explained in Section 1.1, the history of models for light was full of controversy and the currently accepted models are still undergoing change. By contrast, the understanding of sound as wave motion dates to the seventeenth century and has advanced steadily with significant contributions by many physicists and mathematicians.
During the twentieth century, physicists have set themselves the goal of explaining all macro-domain phenomena, including perhaps even life, in terms of these particles, which are called atoms or molecules. Great strides in formulating these explanations have been made, except in the case of phenomena involving the gravitational field. Even Einstein, who reformulated the gravitational interaction in a very novel and general way in his general theory of relativity, was not able to relate gravitation effectively to the electric, magnetic and other fields. One of the most notable areas of progress has been in the invention of models for atoms themselves. Instead of being conceived of as simple point-like particles with no internal structure, atoms are now viewed as complex systems composed of simpler constituents. The properties of atoms are explained in terms of the arrangement and motion of the constituents. In this chapter we will review some of the studies of the current century that have led to the presently accepted models for atoms.
Your common-sense notion of energy is derived from certain systems (bent bows, candle-oxygen systems) that can act as energy sources. In Chapter 4, we identified several forms of energy storage, such as the elastic energy of the bow and the chemical energy of the candle-oxygen system. We also pointed out that a system stores a quantity of energy that depends on the state of the system. In this chapter, we will introduce two operational definitions of energy
Have you ever burned your tongue on hot apple pie? If so, you have probably also noticed that, while you must be careful about the apple filling, the crust is not dangerous even when the pie has just come out of the oven. Visitors to the San Francisco Bay region know the remarkably mild climate enjoyed by the environs of the Bay, where the summer temperature is often 20 or more degrees less than it is only a few miles inland. Both the apple pie effect and the San Francisco climate are related to the fact that water is different from most other materials in its capacity to store thermal energy. All substances become colder or hotter as they lose or gain thermal energy (assuming they are not changing phase, such as in melting or boiling). For water, however, the temperature change is relatively small for a given energy change. The wet pie filling, therefore, transfers energy to the tongue and still remains hot, while the crust cools off substantially when it interacts with the tongue. Conversely, both land and water receive similar amounts of energy from the sun in the summer, but San Francisco Bay water rises in temperature much less than does the adjacent land.
The word “force” is used in everyday language to signify compulsion, either physical or mental. In scientific use, the word “force” has a narrow and quite specific meaning distinct from its everyday meaning. Rather than attempting to present a concise formal definition of force, we will use examples to show how the concept of force grows out of the more general concepts of interaction and energy.
Have you ever burned your tongue on hot apple pie? If so, you have probably also noticed that, while you must be careful about the apple filling, the crust is not dangerous even when the pie has just come out of the oven. Visitors to the San Francisco Bay region know the remarkably In this chapter we will describe some of the conditions under which electric interactions transfer energy in technologically useful ways. To help you with visual images of the process, we will construct an analogue model and a micro-domain model for electrical phenomena. To help you make mathematical models for the process, we will introduce two variable factors describing it: the magnitude of the electric current, and the voltage of the energy source. These two variables can be ex- plained qualitatively by applying them to an electric spark. The bright- ness of the spark is related to the magnitude of the current, while the distance the spark jumps is related to the voltage of the energy source.
In this chapter, we will introduce a new concept, the velocity of a moving object, which takes into account the direction of motion as well as the speed. Like position, displacement, and force, velocity will be denoted by a boldface letter in the text (s, ∆s, F, v) and by an arrow in a diagram. The operations of arithmetic apply to velocity in the same way as to displacements.
Newton’s laws lead to mathematical models for the relation among force, inertial mass, acceleration, and momentum. These models will enable you to predict the motion of bodies that are subject to known forces and to make inferences about the forces from observed motion.
Periodic motion can occur in a system only when the objects in the system interact with one another in such a way that they remain near one another. In the absence of interaction, the objects would move apart and not return to repeat their motion once they had passed each other. Even though systems carrying out periodic motion will eventually stop moving, they do have a stable existence over many cycles of their motion, as shown by the example of the solar system. The gasoline engine in automobiles, motorcycles, lawnmowers, and other similar appliances is one of the major conveniences of modern technology, but it is also, because of the air pollution it causes, one of the major threats to civilization. The gasoline engine, diesel engine, and gas turbine jet engine are modern counterparts of the coal-burning steam engine, whose development by James Watt (1736-1819) led to the industrial revolution in the nineteenth century. All of them trans- form heat released by burning fuel into useful work for manufacturing, construction, and transportation; they are special types of what are called heat engines. Read more by clicking the button below.
The gasoline engine in automobiles, motorcycles, lawnmowers, and other similar appliances is one of the major conveniences of modern technology, but it is also, because of the air pollution it causes, one of the major threats to civilization. The gasoline engine, diesel engine, and gas turbine jet engine are modern counterparts of the coal-burning steam engine, whose development by James Watt (1736-1819) led to the industrial revolution in the nineteenth century. All of them trans- form heat released by burning fuel into useful work for manufacturing, construction, and transportation; they are special types of what are called heat engines. Read more by clicking the button below.
About the Authors
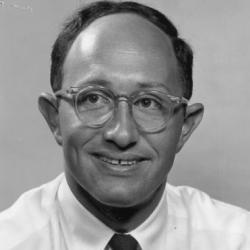
Robert Karplus
Robert Karplus (1927–90), who began his career as a brilliant theoretical physicist, switched to science education in the early 1960s. He made many substantial contributions to this field in addition to developing a complete K–6 hands-on science curriculum. Karplus provided his curriculum with a sound epistemological foundation, based on the work of Piaget. He developed an effective classroom teaching strategy, the learning cycle. He and his team used a scientific approach to curriculum development. They focused on teacher development. Karplus was committed to science for ALL students. Through science activities he sought to share the joy of discovery.
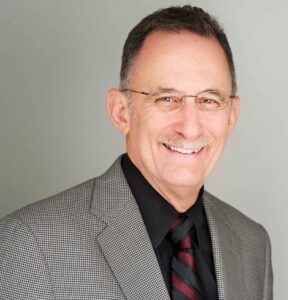
Fernand Brunschwig
Fernand Brunschwig has been teaching ever since his high school history teacher occasionally handed over the chalk. Then he tutored a student who had failed miserably at her chemistry final exam in June, and she blasted it out of the park in September. Concluding that this was a fun way to earn a living, Fernand decided to teach physics rather than pursue research in 1964 after his undergrad degree at Harvard. He taught PSSC Physics as an intern and earned an MAT at Harvard and then taught PSSC Physics, ChemStudy Chemistry, Intro Physical Science (IPS), and middle school science for 3 years at American schools in Europe before returning to the US and earning a Master’s in Physics and a PhD in Science Education with Robert Karplus at Berkeley in 1972. Fernand then joined SUNY Empire State College as a member of the founding faculty and retired in 2012 after much successful innovation. He had added an MS in computer science at CCNY in 1987. A part-time position teaching physics to graduate students at Teachers College led to involvement with a group of TC graduates/physics teachers in 2011 who formed a support group for physics teachers, called “PhysicsTeachersNYC,” which Fernand agreed to lead. The idea took off, and the group became STEMteachersNYC, which has grown to over 1400 members throughout the NY Metro Area and has evolved into today’s thriving non-profit devoted to professional development for teachers, by teachers, about teaching.
This work is licensed under a Creative Commons Attribution-NonCommercial-ShareAlike 4.0 International License.